Innovative heat recovery project for carbon emissions reduction
Vornado Realty Trust is spearheading a groundbreaking retrofit at 1 Pennsylvania Plaza (PENN 1) in New York City, aiming to reach 100% carbon neutrality by 2040. This ambitious project is part of the company’s broader commitment to environmental sustainability, as outlined in their Vision 2030. PENN 1, a towering landmark in midtown Manhattan, stands at 57 stories and spans approximately 2.5 million square feet of office and retail space. Constructed in 1972, the building is a key component of THE PENN DISTRICT, Vornado’s flagship property cluster.
The roadmap to carbon neutrality at PENN 1 includes advanced waterside heat recovery measures. This strategy focuses on capturing and reusing heat from the building’s condenser water loop, a method that not only reduces heating loads but also facilitates a subsequent transition to electrification through air-source heat pumps and thermal storage. The PENN 1 project demonstrates a ‘thermal dispatch model,’ in which carbon-free energy sources are gradually deployed to fulfill the heating and cooling demands of a large commercial building.
By adopting this innovative approach, Vornado aims to significantly reduce the carbon footprint of PENN 1 while also setting a replicable model for building decarbonization in New York City. This initiative underscores Vornado’s role as a leader in sustainable real estate development, with a portfolio that includes over 34 million square feet of premier assets across New York City, Chicago, and San Francisco. Through Vision 2030, Vornado’s commitment to achieving carbon neutrality and a 50% site energy reduction reflects their dedication to pioneering sustainable solutions in the urban landscape.
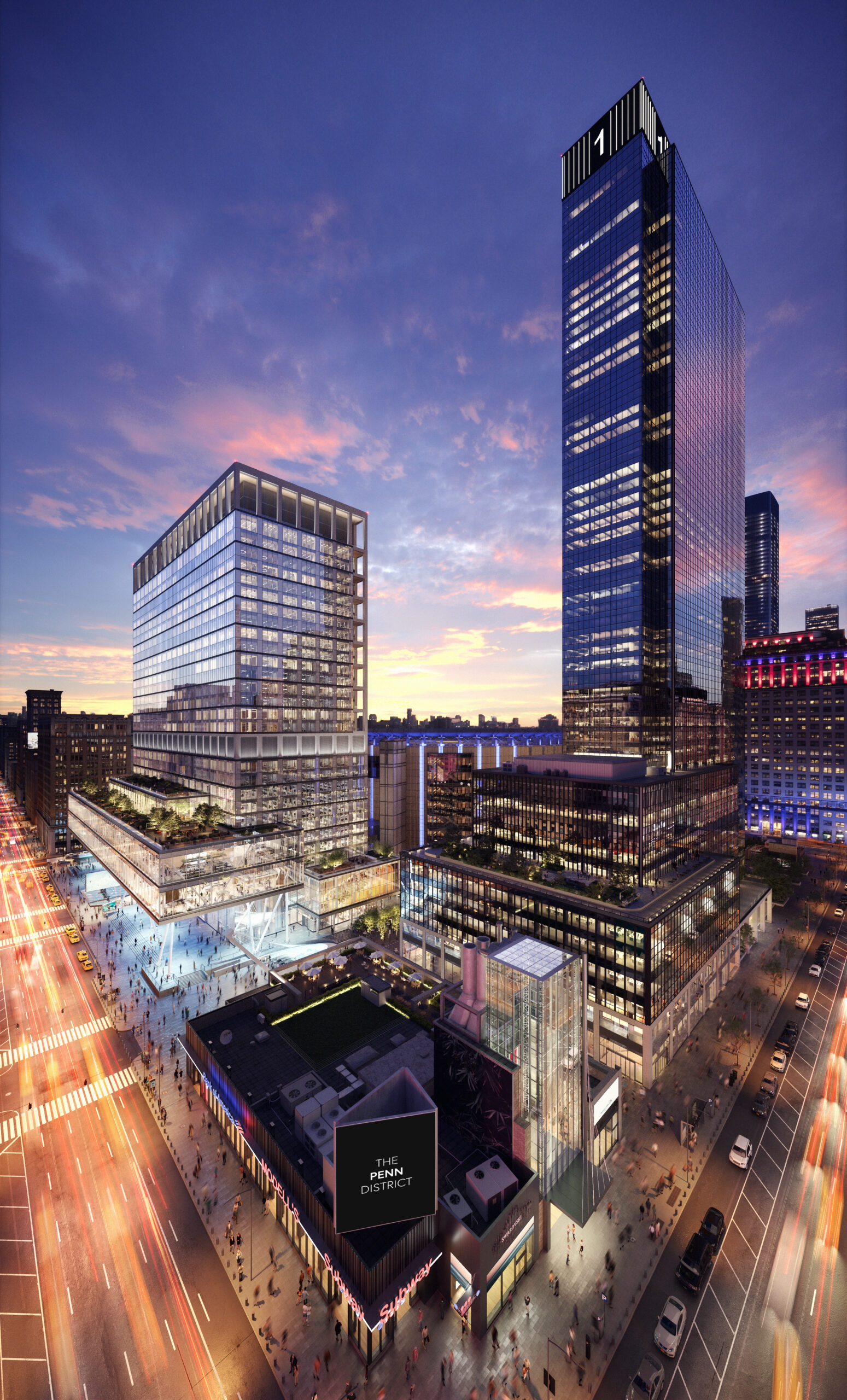
Project Status
Planning
Under Construction
Monitoring & Evaluation
Project Highlights
Step 1
Step 1: Examine Current Conditions
A baseline assessment is key to understanding current systems and performance, then identifying conditions, requirements or events that will trigger a decarbonization effort. The assessment looks across technical systems, asset strategy and sectoral factors.
Building System Conditions
- Equipment nearing end-of-life
- New heat source potential
- Tenant load change
- Resilience upgrades
- Efficiency improvements
Asset Conditions
- Repositioning
- Capital event cycles
- Carbon emissions limits
- Tenant sustainability demands
- Investor sustainability demands
Market Conditions
- Technology improves
- Policy changes
- Utility prices change
- Fuels phase out
Step 2
Step 2: Design Resource Efficient Solutions
Effective engineering integrates measures for reducing energy load, recovering wasted heat, and moving towards partial or full electrification. This increases operational efficiencies, optimizes energy peaks, and avoids oversized heating systems, thus alleviating space constraints and minimizing the cost of retrofits to decarbonize the building over time.
Existing Conditions
This diagram illustrates the building prior to the initiation of Strategic Decarbonization planning by the owners and their teams.
Click through the measures under “Building After” to understand the components of the building’s energy transition.
Sequence of Measures
2022
2023
2024
2025
2030
Building System Affected
- heating
- cooling
- ventilation
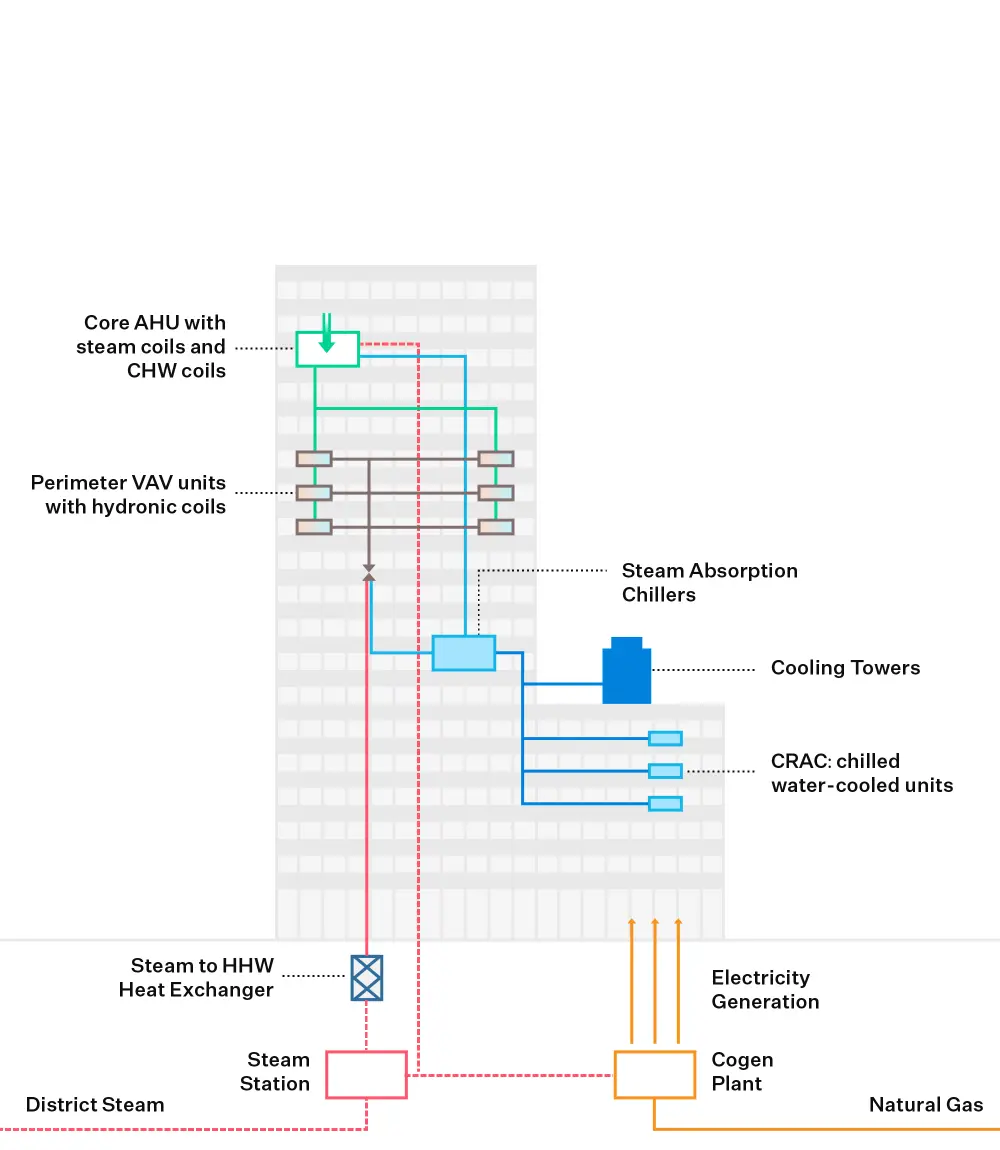
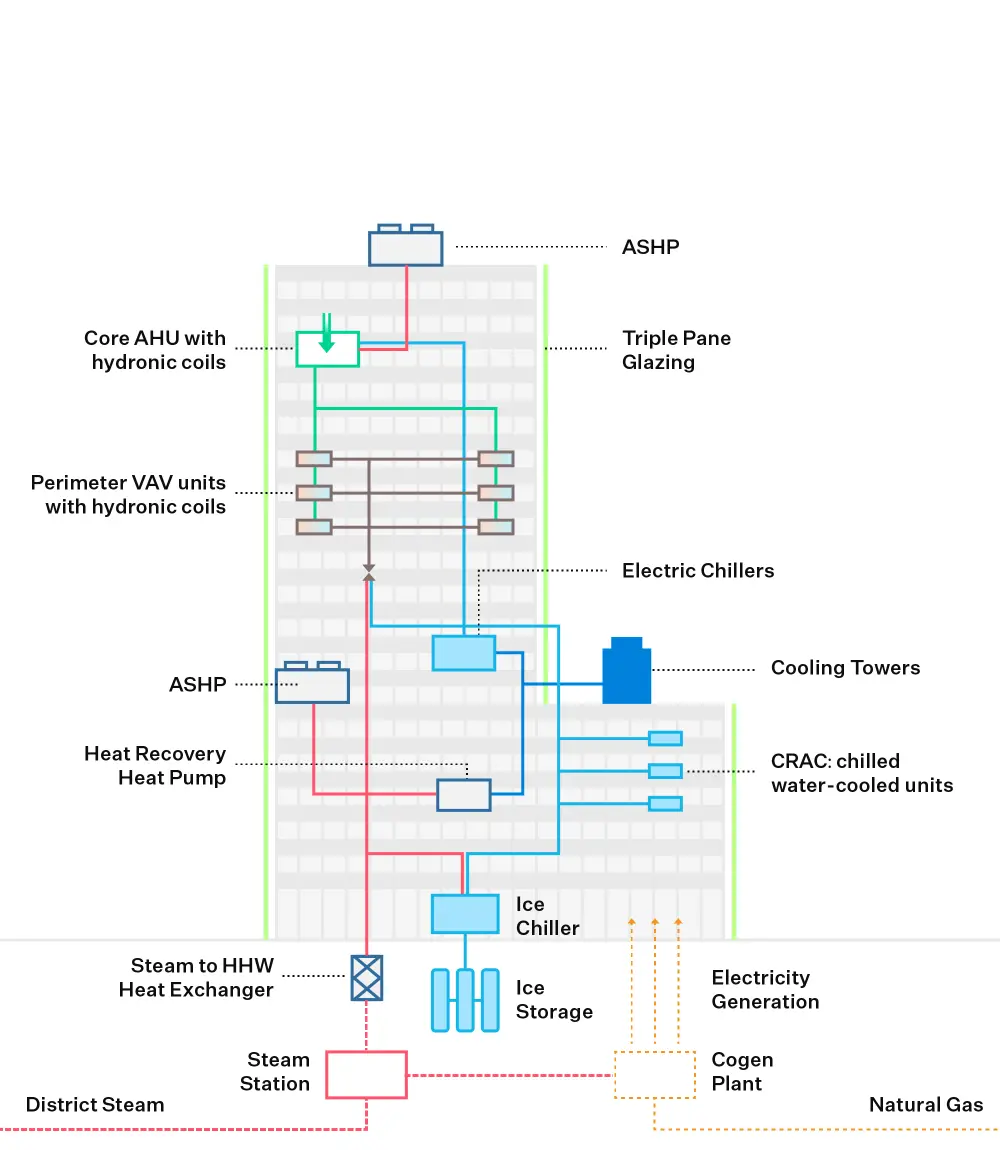
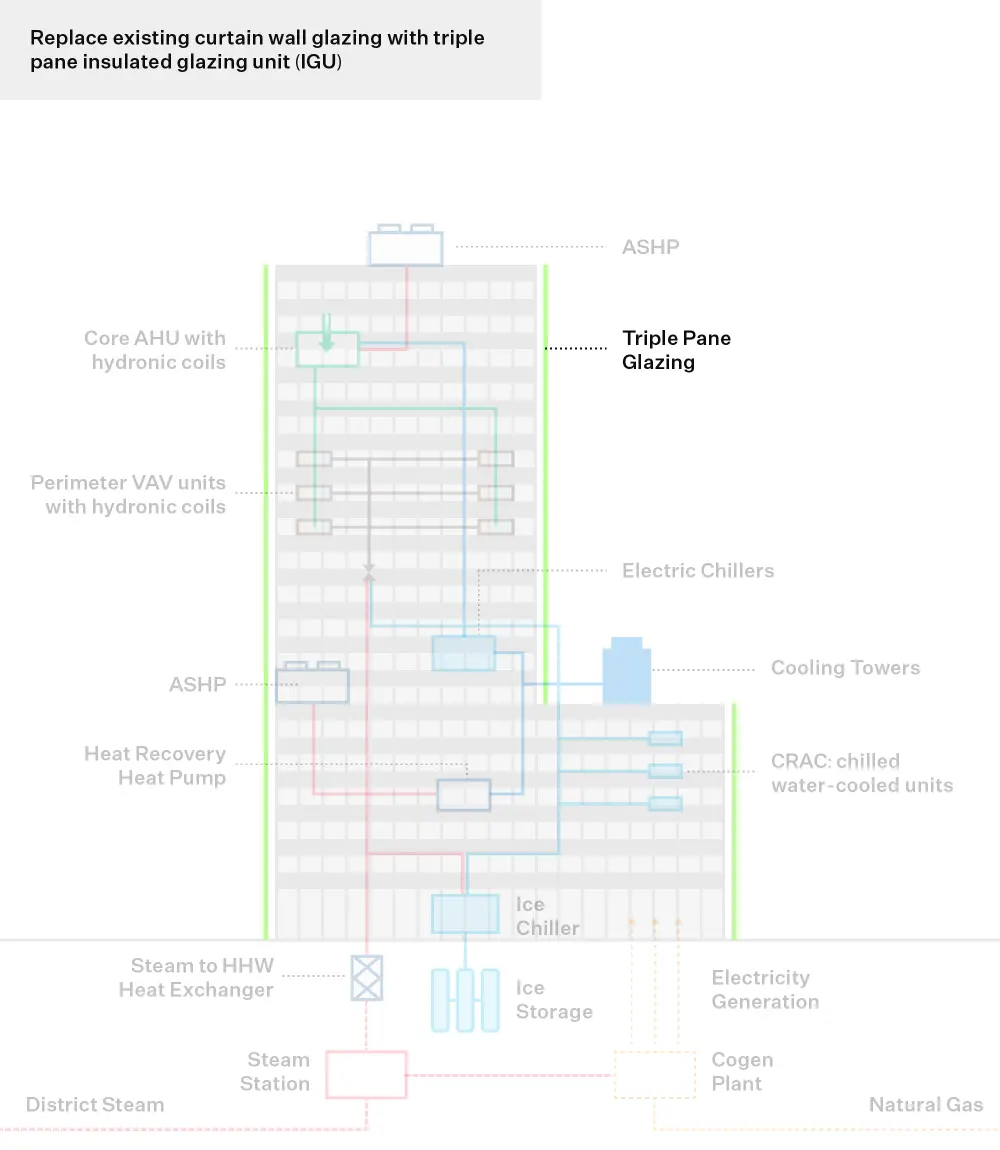
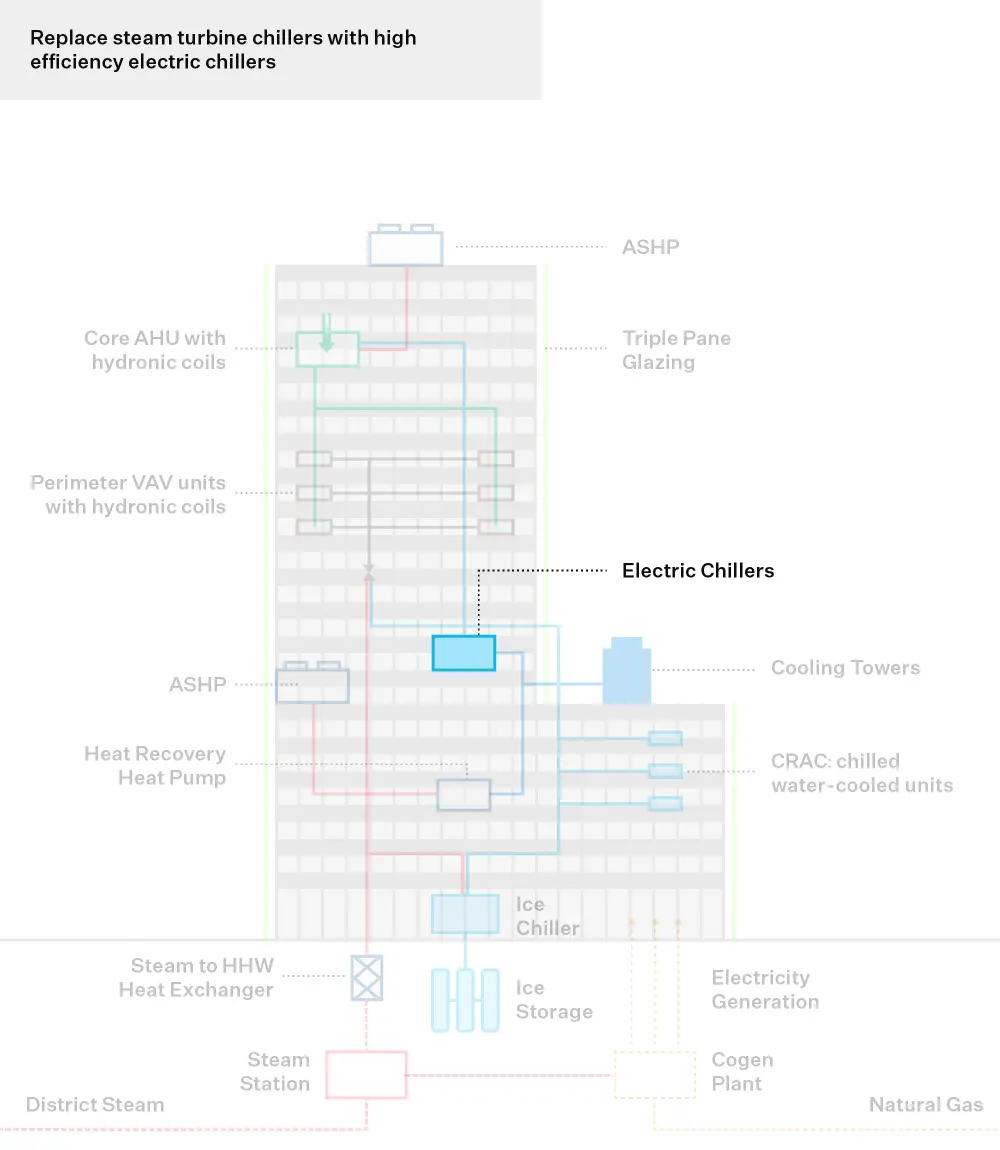
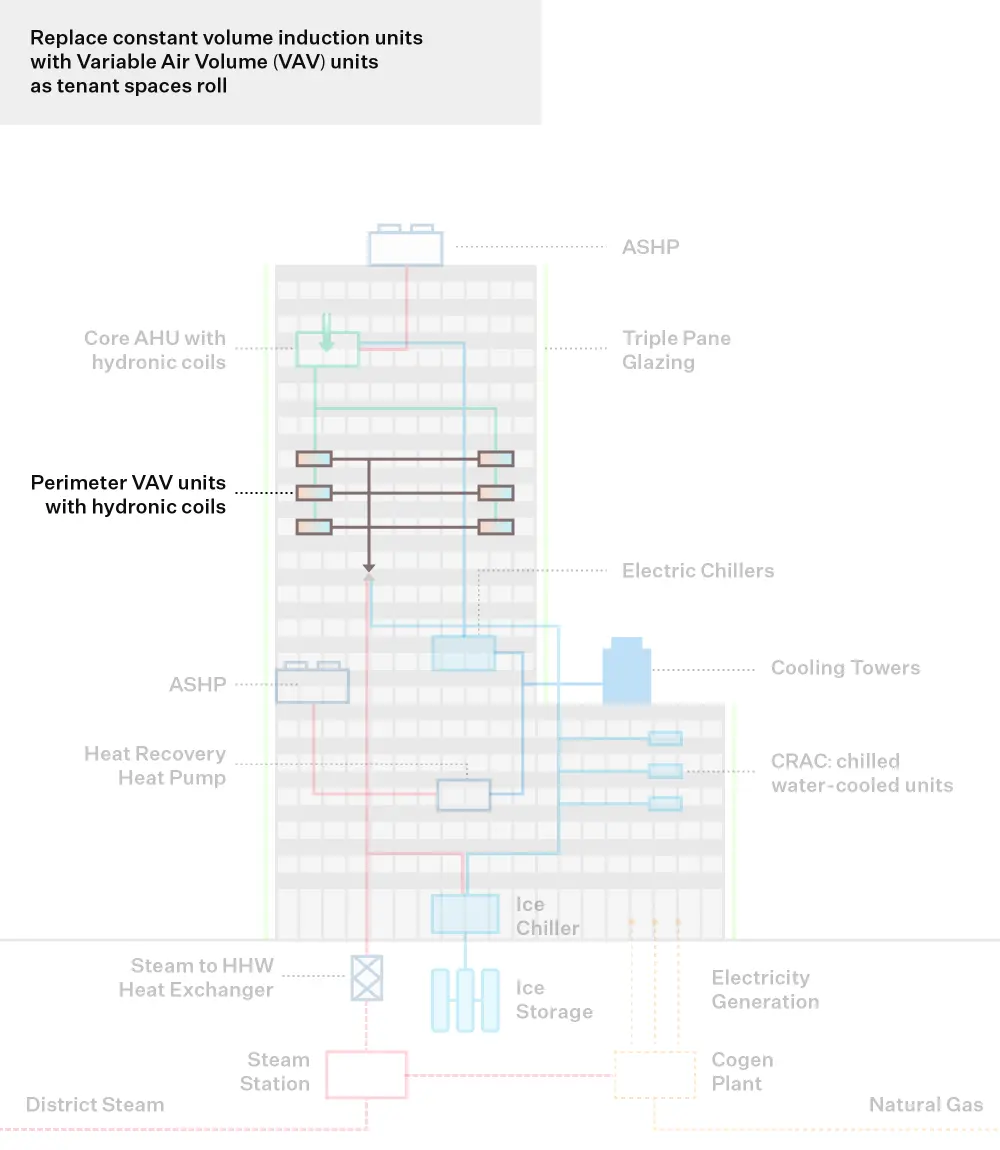
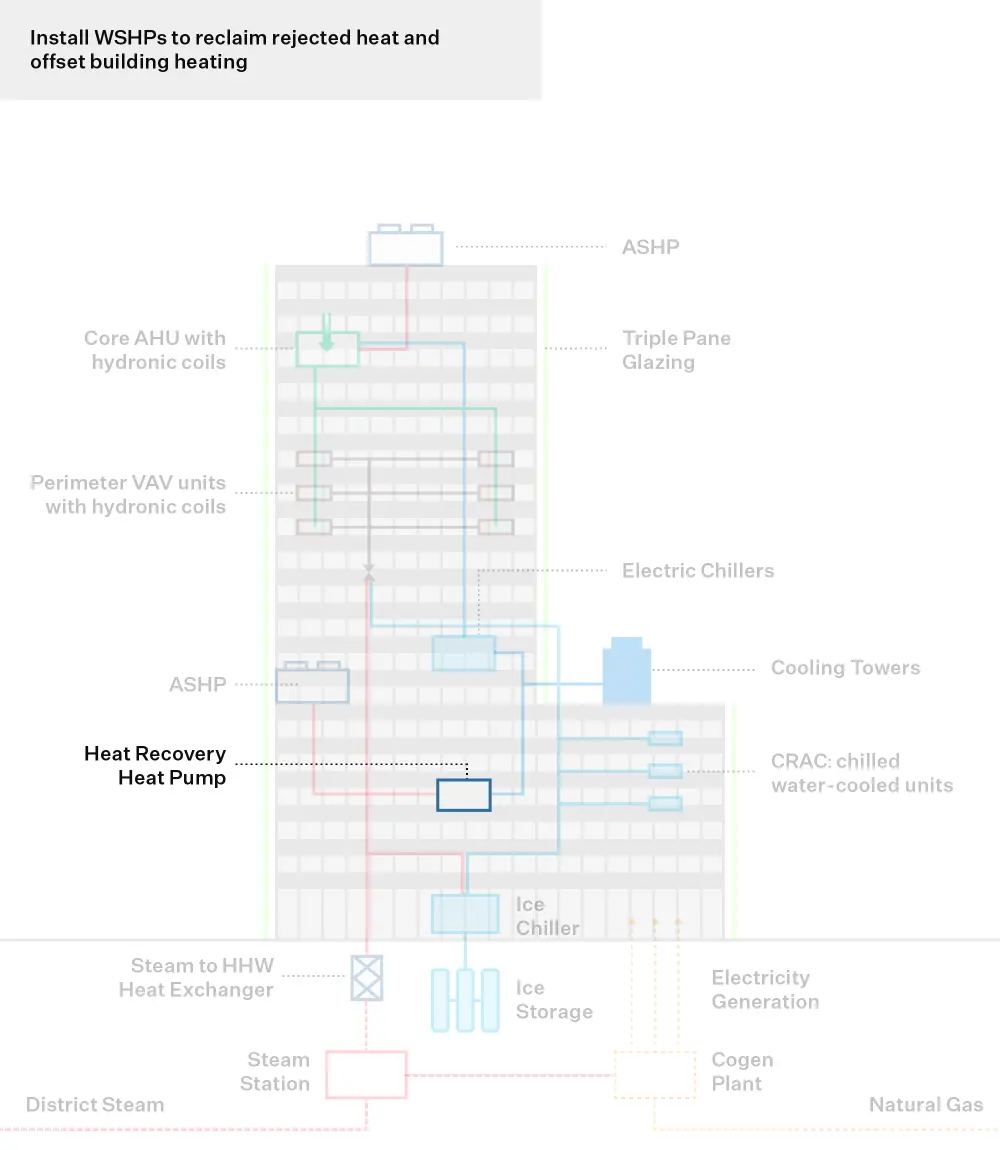
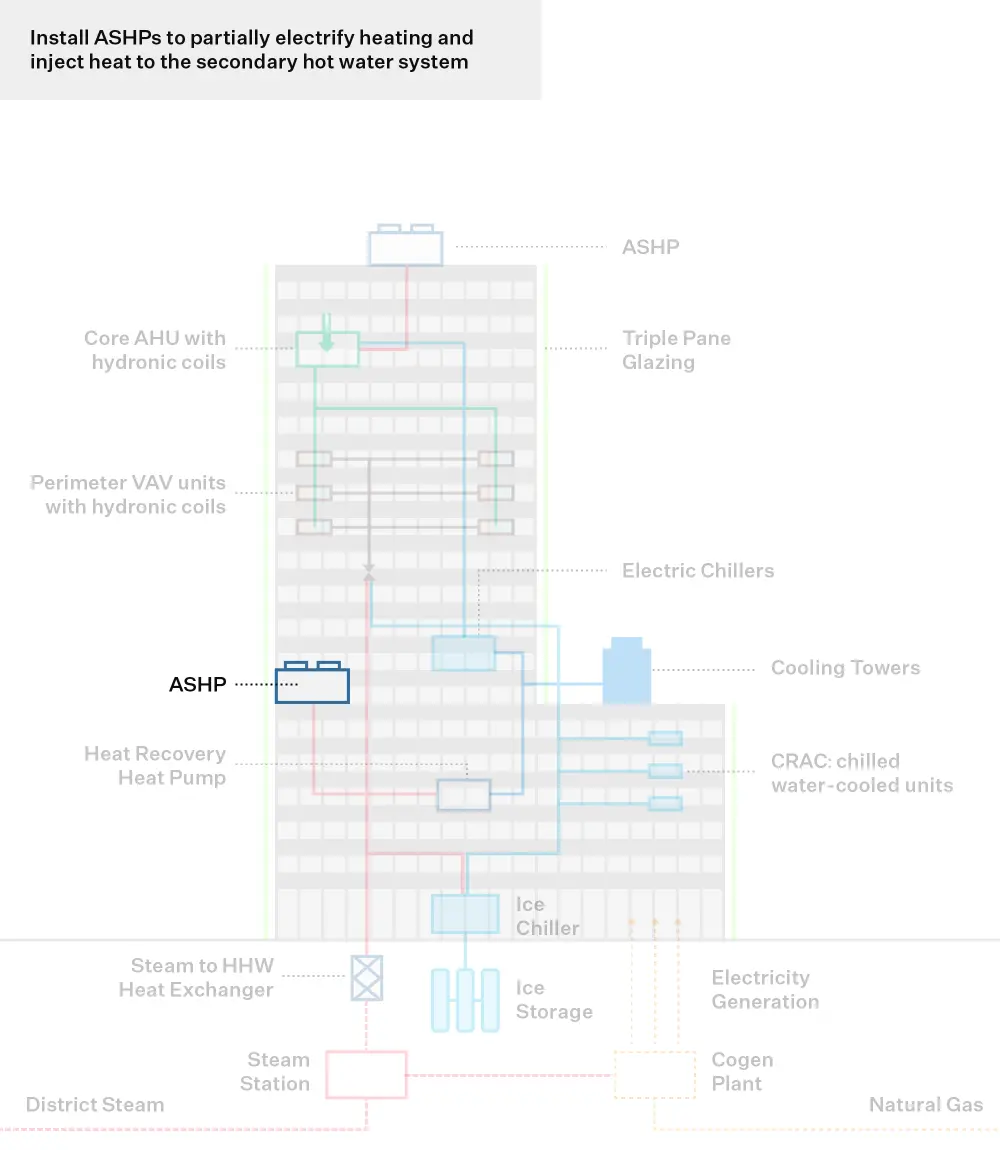
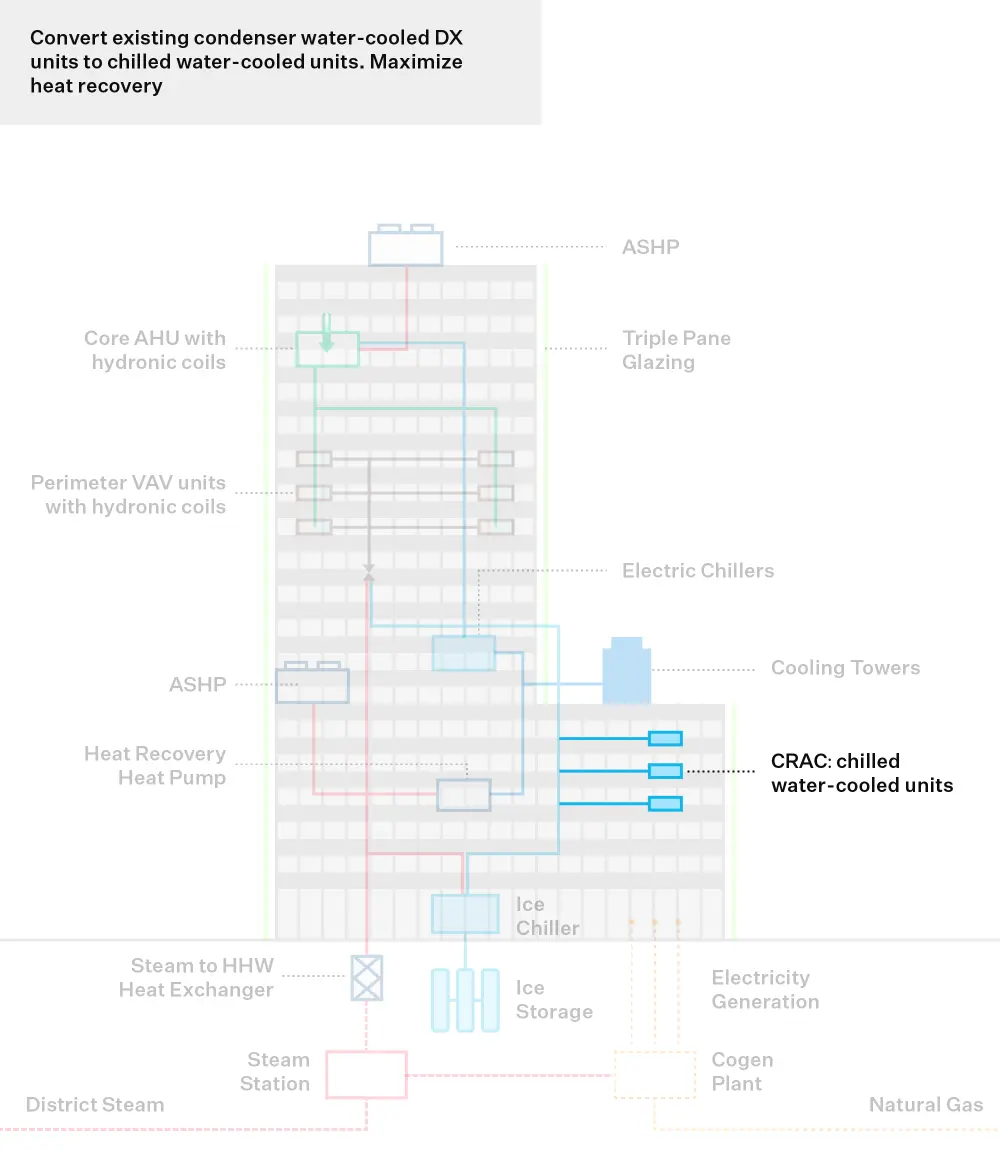
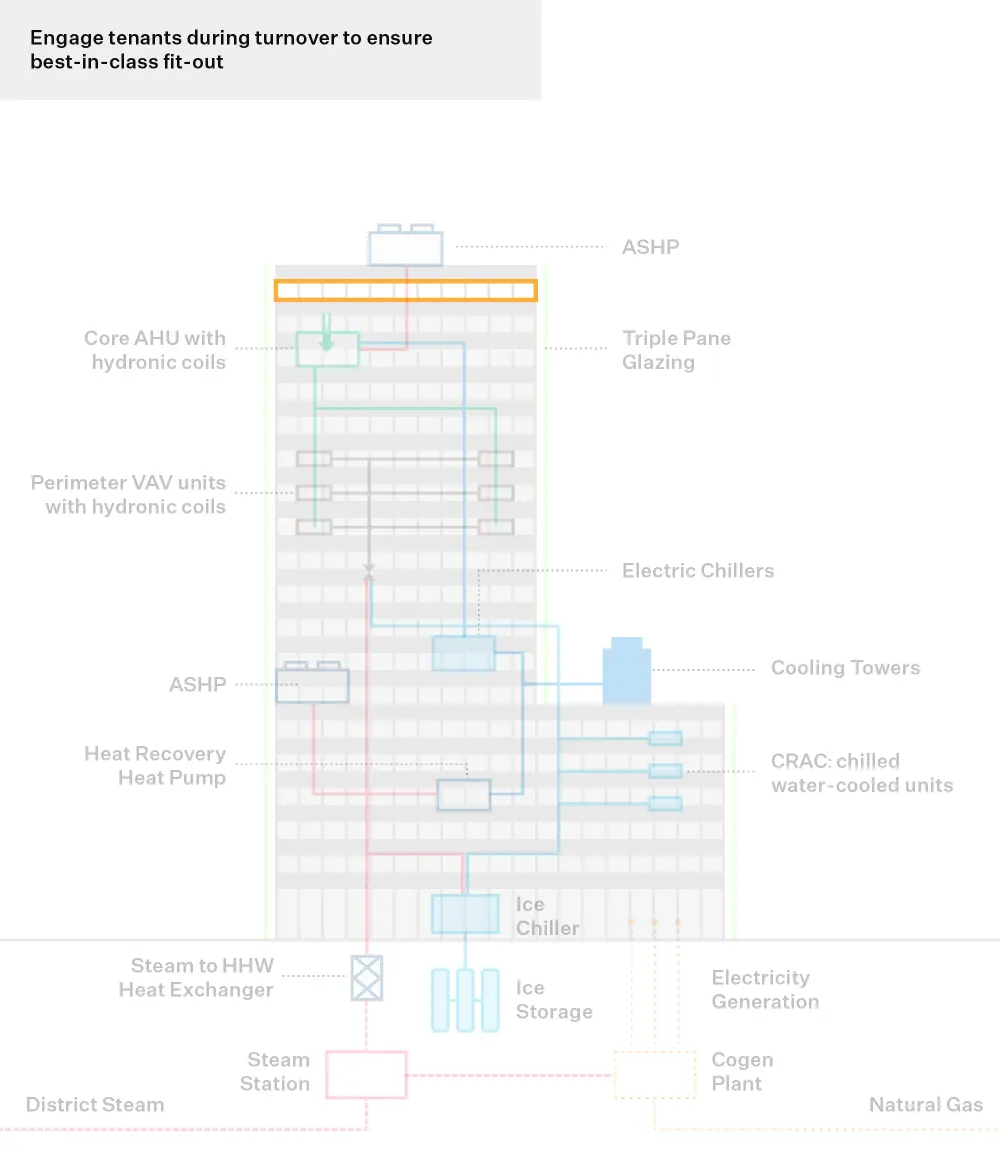
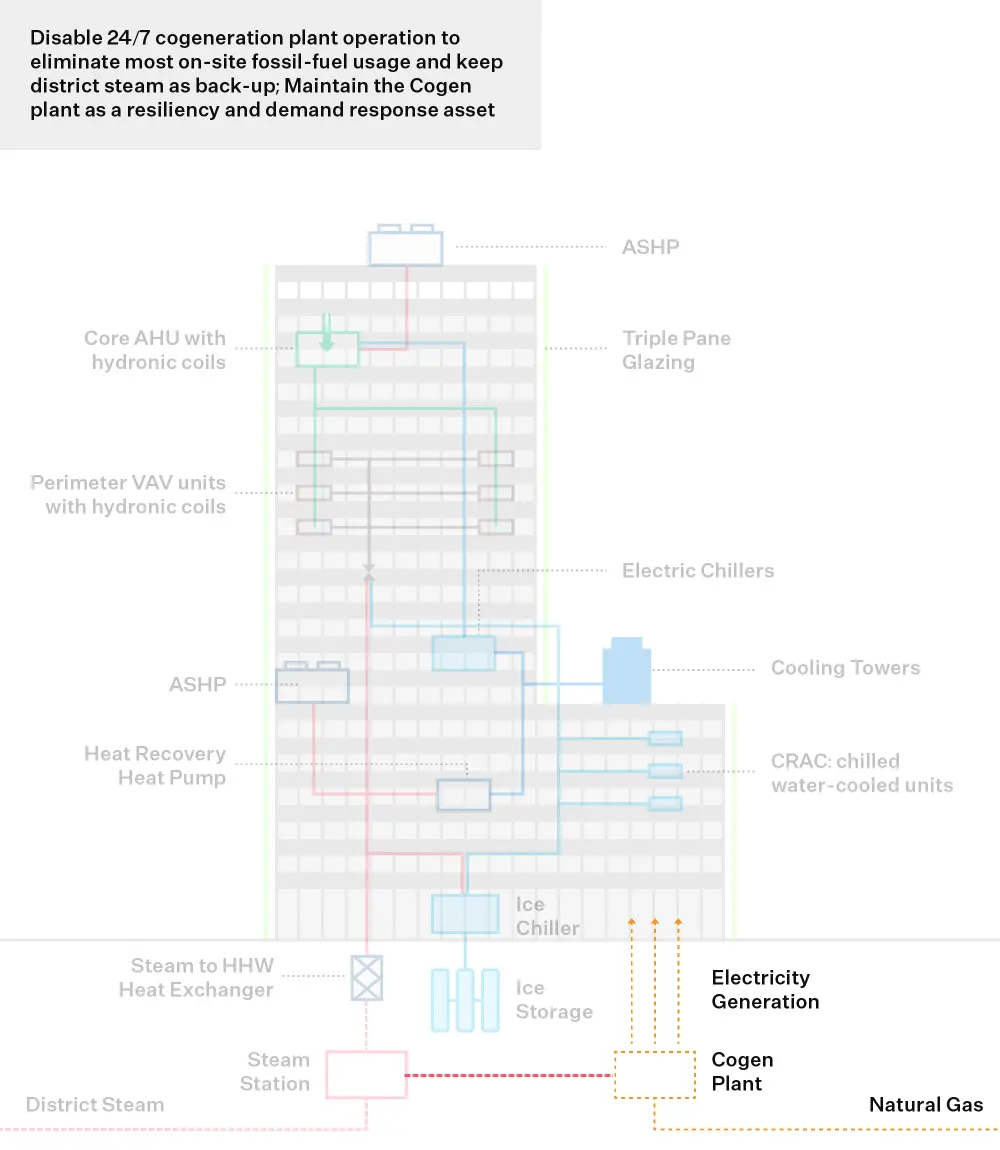
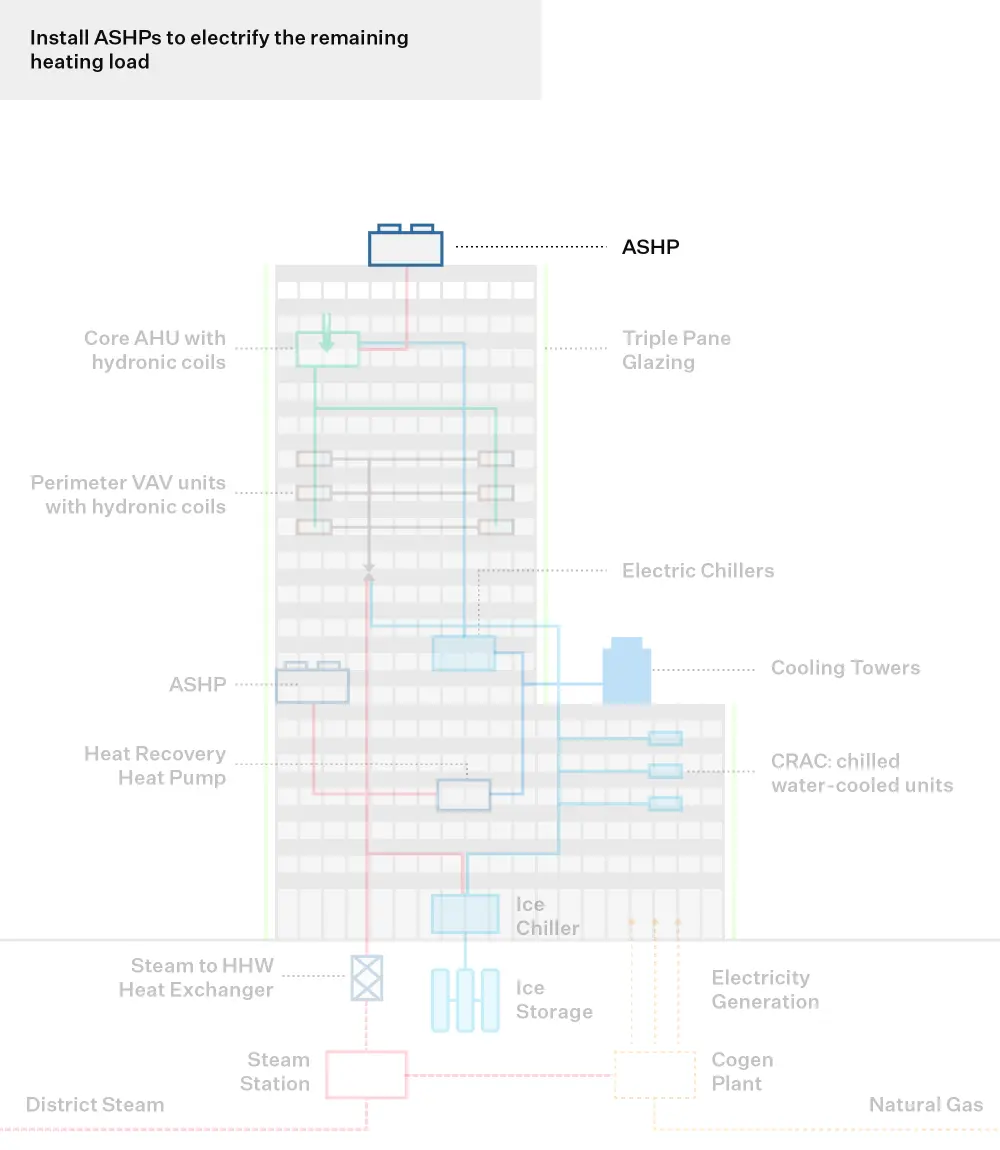
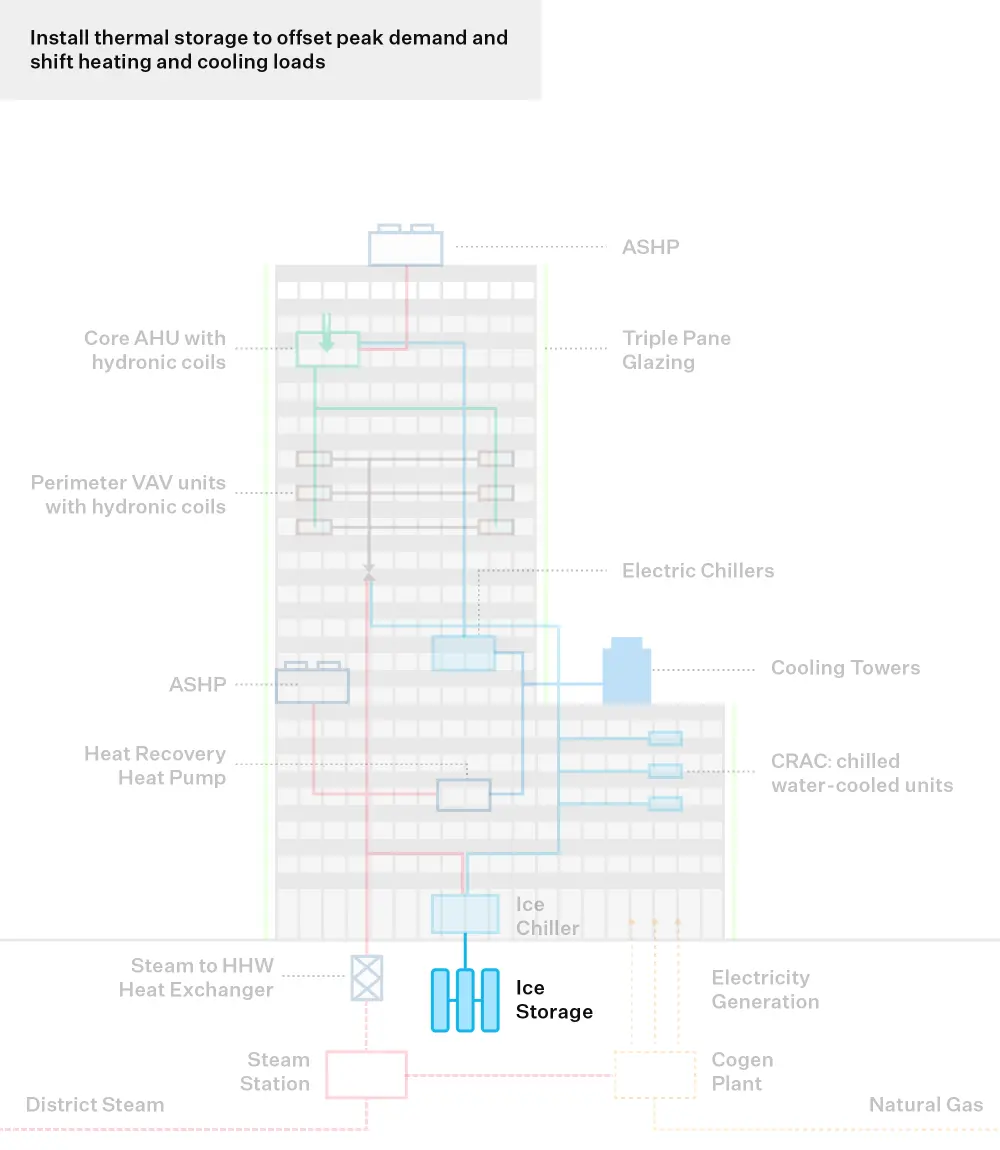
Step 3
Step 3: Build the Business Case
Making a business case for strategic decarbonization requires thinking beyond a traditional energy audit approach or simple payback analysis. It assesses business-as-usual costs and risks against the costs and added value of phased decarbonization investments in the long-term.
Strategic Decarbonization Action Plan
An emissions decarbonization roadmap helps building owners visualize their future emissions reductions by outlining the CO2 reductions from selected energy conservation measures. This roadmap is designed with a phased approach, considering a 20- or 30-year timeline, and incorporates the evolving benefits of grid decarbonization, ensuring a comprehensive view of long-term environmental impact.
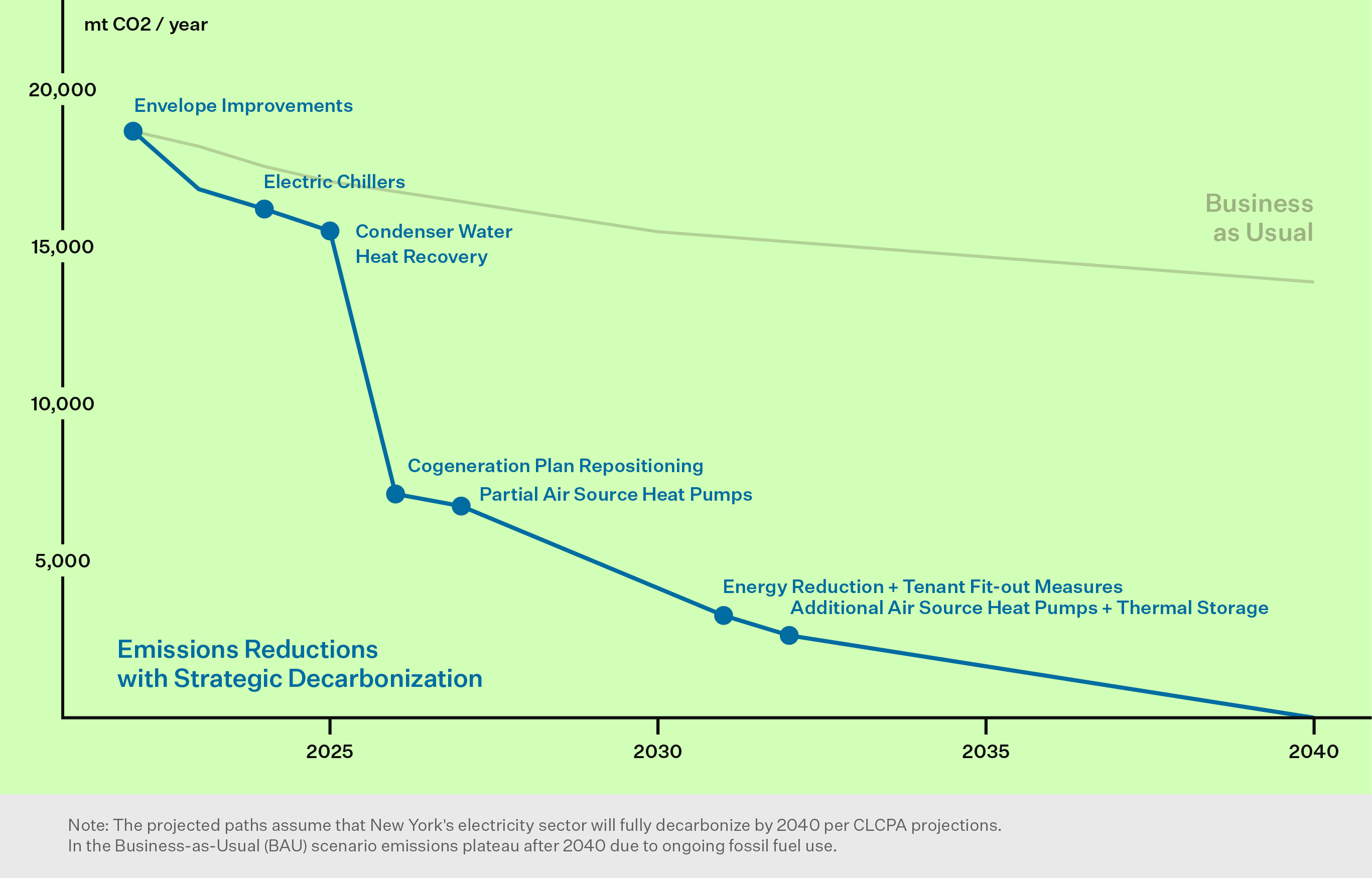